CHF (Critical Heat Flux)
During the brainstorming session, we have come with a critical question on ‘Furnace life’ due to higher heat loading in the furnace. during the ‘research’, I came across a term, Critical heat flux(CHF) or the ‘burn out point’.
The past decade has witnessed unprecedented improvements in the performance of packaged boiler which were brought about, for the most part, by a restless pursuit of reducing the foot print of boiler. These advances have led to increases in the amount of heat that is dissipated and has to be removed from these furnaces to keep its life up & good heat transfer, large increase in heat dissipation per unit surface area is now the benchmark for designing of the furnace.
The CHF is a very interesting and important phenomenon from both fundamental and practical points of view. From the fundamental point of view, CHF accompanies tremendous changes in heat transfer, pressure drop and flow regime.
The critical heat flux (CHF) condition is characterized by a sharp reduction of the local heat transfer coefficient that results from the replacement of liquid by vapor adjacent to the heat transfer surface (Collier & Thome, 1994). The occurrence of CHF is accompanied by an inordinate increase in the surface temperature for heat-flux-controlled systems, and an inordinate decrease in the heat transfer rate for temperature-controlled systems. The CHF condition is generally more important in the heat-flux-controlled systems, since the temperature increase can threaten the physical integrity of the heated surface.



Dissipation of large heat fluxes at relatively small temperature differences is possible in systems utilizing boiling phenomenon as long as the heated wall remains wetted with the liquid. With the wetted wall condition at the heated surface, heat is transferred by a combination of two mechanisms:
(i) bubbles are formed at the active nucleation cavities on the heated surface, and heat is transferred by the nucleate boiling mechanism, and
(ii) heat is transferred from the wall to the liquid film by convection and goes into the bulk liquid or causes evaporation at the liquid-vapor interface. The large amount of energy associated with the latent heat transfer (compared to the sensible energy change in the liquid corresponding to the available temperature potential in the system) in the case of nucleate boiling, or the efficient heat transfer due to liquid convection at the wall, both lead to very high heat transfer coefficients in flow boiling systems. Removal or depletion of liquid from the heated wall therefore leads to a sudden degradation in the heat transfer rate.
The way in which the heated surface arrives at the liquid starved condition in a flow boiling system determines whether it is termed as Critical Heat Flux
At atmospheric pressure, The critical heat flux is slightly above 1MW/m². The formula for calculation of heat flux is given below.
This formula is derived by Zuber,N and its more in line with the practical values
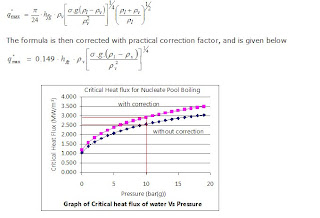


Superheater Program
See the attached screen shot of the program.
It includes all possible configuration of the Super-hater.
The program is for Convective super-heater.
Main Window
The Super heater module